
漢德百科全書 | 汉德百科全书
3D printing has already proven its worth in the plastic industry and grows further in terms of innovative processes, use of tricky polymers and different temperature ranges. The new methods and material are helping in developing plastic parts or objects cost-efficiently and rapidly.
This channel contains the recent advances in a new method and machine development, process optimization and product development (Filaments, 3D Printable Resins and Polymer Powders, and Sustainable Polymers apt for 3D printing). You may also find the breakthrough research projects that are developing plastic parts with challenging designs and materials.
Get industry updates related to 3D printing, additive manufacturing and 4D printing directly in your mailbox every month. Subscribe to this channel for free and stay up to date with recent advances. (Quelle:https://omnexus.specialchem.com/channel/3d-printing)
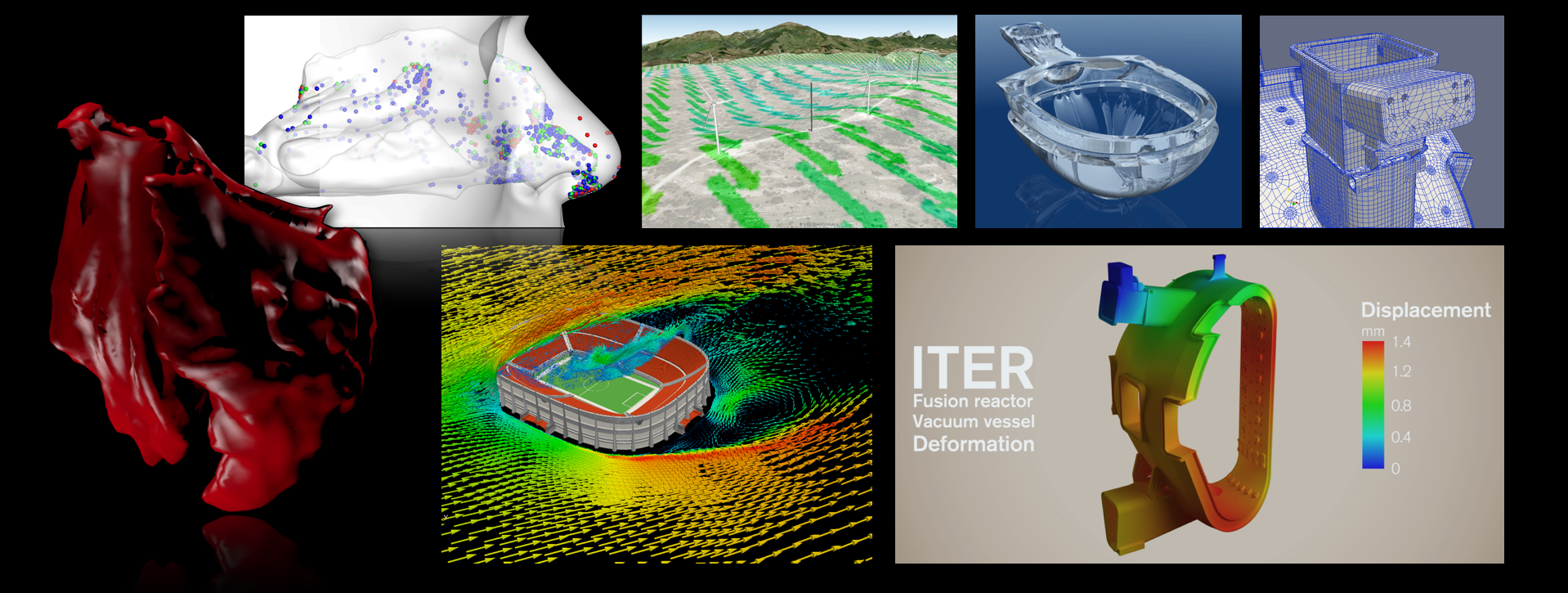
Supraleiter sind Materialien, deren elektrischer Widerstand beim Unterschreiten der sogenannten Sprungtemperatur praktisch Null wird. Die Supraleitung wurde 1911 von Heike Kamerlingh Onnes, einem Pionier der Tieftemperaturphysik, entdeckt. Sie ist ein makroskopischer Quantenzustand.[1]
Viele Metalle, aber auch andere Materialien sind Supraleiter. Die Sprungtemperatur – auch „kritische Temperatur“ Tc genannt – ist für die meisten Supraleiter sehr niedrig; um Supraleitung zu erreichen, muss das Material im Allgemeinen mit verflüssigtem Helium (Siedetemperatur −269 °C) gekühlt werden. Nur bei den Hochtemperatursupraleitern genügt zur Kühlung verflüssigter Stickstoff (Siedetemperatur −196 °C).
Im supraleitenden Zustand tritt der Meißner-Ochsenfeld-Effekt auf, d. h. das Innere des Materials bleibt bzw. wird frei von elektrischen und magnetischen Feldern. Ein elektrisches Feld würde durch die ohne Widerstand beweglichen Ladungsträger sofort abgebaut. Magnetfelder werden durch den Aufbau entsprechender Abschirmströme an der Oberfläche verdrängt, die mit ihrem eigenen Magnetfeld das von außen eindringende Magnetfeld kompensieren. Ein nicht zu starkes Magnetfeld dringt nur etwa 100 nm weit in das Material ein; diese dünne Schicht trägt die Abschirm- und Leitungsströme.
Der Stromfluss durch den Supraleiter senkt die Sprungtemperatur. Die Sprungtemperatur sinkt auch, wenn ein äußeres Magnetfeld anliegt. Überschreitet das Magnetfeld einen kritischen Wert, so beobachtet man je nach Material verschiedene Effekte. Bricht die Supraleitung schlagartig zusammen, spricht man von einem Supraleiter erster Art oder vom Typ I. Supraleiter zweiter Art dagegen (Typ II) haben zwei kritische Feldstärken, ab der niedrigeren beginnt das Feld einzudringen, bei der höheren bricht die Supraleitung zusammen. In dem Bereich dazwischen dringt das Magnetfeld in Form mikroskopisch feiner Schläuche zunehmend in den Leiter ein. Der magnetische Fluss in diesen Flussschläuchen ist quantisiert. Supraleiter vom Typ II sind durch ihre hohe Stromtragfähigkeit interessant für technische Anwendungen.
Technische Anwendungen der Supraleitung sind die Erzeugung starker Magnetfelder – für Teilchenbeschleuniger, Kernfusionsreaktoren, Magnetresonanztomographie, Levitation – sowie Mess- und Energietechnik.
超导现象(英語:Superconductivity)是指材料在低于某一温度时,电阻变为零的现象,而这一温度称为超导转变温度(Tc)。超导现象的特征是零电阻和完全抗磁性。
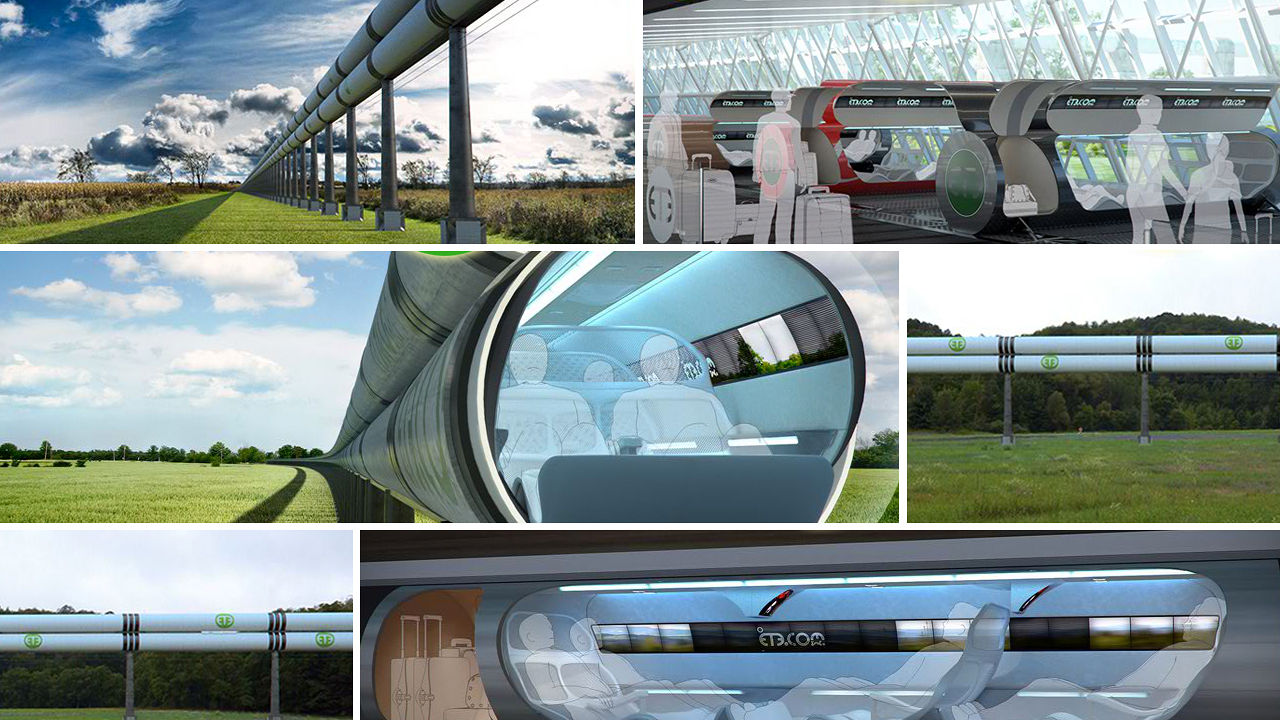
























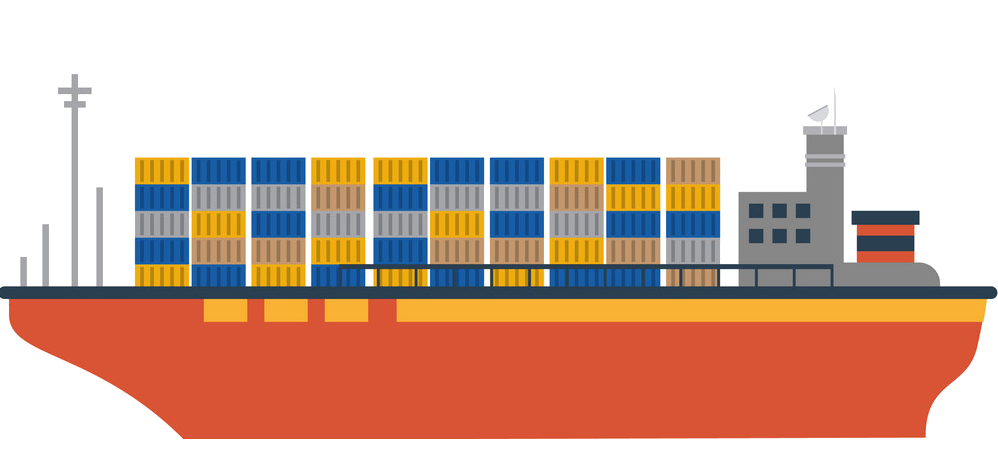



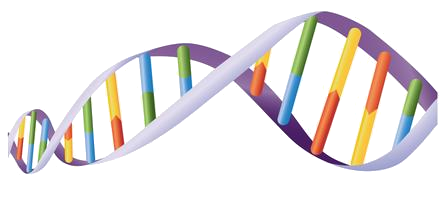

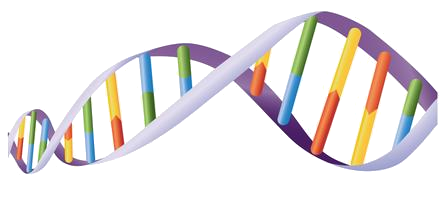

Energiespeicher dienen der Speicherung von momentan verfügbarer, aber nicht benötigter Energie zur späteren Nutzung. Diese Speicherung geht häufig mit einer Wandlung der Energieform einher, beispielsweise von elektrischer in chemische Energie (Akkumulator) oder von elektrischer in potenzielle Energie (Pumpspeicherkraftwerk). Im Bedarfsfalle wird die Energie dann in die gewünschte Form zurückgewandelt. Sowohl bei der Speicherung als auch bei der Energieumwandlung treten immer – meist thermische – Verluste auf.
储能或储能技术指的是把能量储存起来,在需要时使用的技术。储能技术将较难储存的能源形式,转换成技术上较容易且成本低的形式储存起来。例如:太阳能热水器将光能(辐射)存在热水(热能)里,电池将电能存在电化学能里。
一般当可再生能源的发电占比低时(例如20%以下),原有电网中作为尖离峰用电调节的负载追随电厂(例如:燃气发电和水力发电),可应付间歇性再生能源在供电量的变化。然而,当占比高到一定程度,就需要有额外的可以调节系统来维持供电平衡[1]。储能为其中一个重要的技术,另外还有需求侧管理以及电网互连)。
储存能量有许多用途,例如:应急能源,也可以用于在电网负荷低的时候储能,在电网高负荷的时候输出能量,用于削峰填谷,减轻电网波动。储存能量有多种形式,包括机械能、热能、电化学能、化学的及电子[2] 。能量储存涉及将难以储存的形式的能量转换成更便利或经济可存储的形式。 大量储能目前主要由发电水坝组成,无论是传统的还是水泵抽水的。
每种技术适合储存的时间长短不一,例如:热水能存数小时,而氢气储能可存数天至数个月以上。目前大型储能系统主要为水力发电和抽蓄发电。电网储能指的是用在电网的大型储能装置。
储能技术在日常生活中随处可见。蓄电池储存的电化学能,可以转换成电能供应电子产品使用。水库储存了水的重力位能,透过水力发电转换成电能。储冷系统利用离峰的电能制造冰块,储存起来的热能可在尖峰时使用降低制冷的用电。生物借由吸收太阳能生长,死亡后长期埋在地底下,转变后成为化石燃料。食物储存了化学能,经过消化吸受后可供应人体热量。
在20世纪,电力系统主要是靠燃烧化石燃料来发电。当用电量改变时,发电量可透过减少燃料使用来调整。近年来,因为空气污染、进口能源依赖及全球暖化等议题,使得再生能源(如:风能及太阳能)快速的发展[3]。然而,风力发电无法控制,发电时不一定在需要用电的时候。太阳能发电会受到云的遮蔽影响,且只有白天才能发电,无法供应晚上的尖峰用电(请参考鸭子曲线)。因此,随着再生能源的发展,能把间歇性能源存起来的技术,越来越受到重视[4][5][6]。
21世纪以来,移动设备使用快速增加,使得电池相关的使用更普及。在全球偏远的地区,太阳能的使用越来越普遍[7]。是否有电能用,不再有技术上的限制,而是经济和财务上的问题[8]。随着电动车普及,短距离交通运输可不再依赖化石燃料,但在长距离运输(如:空运和海运)方向,相关技术还在发展中。











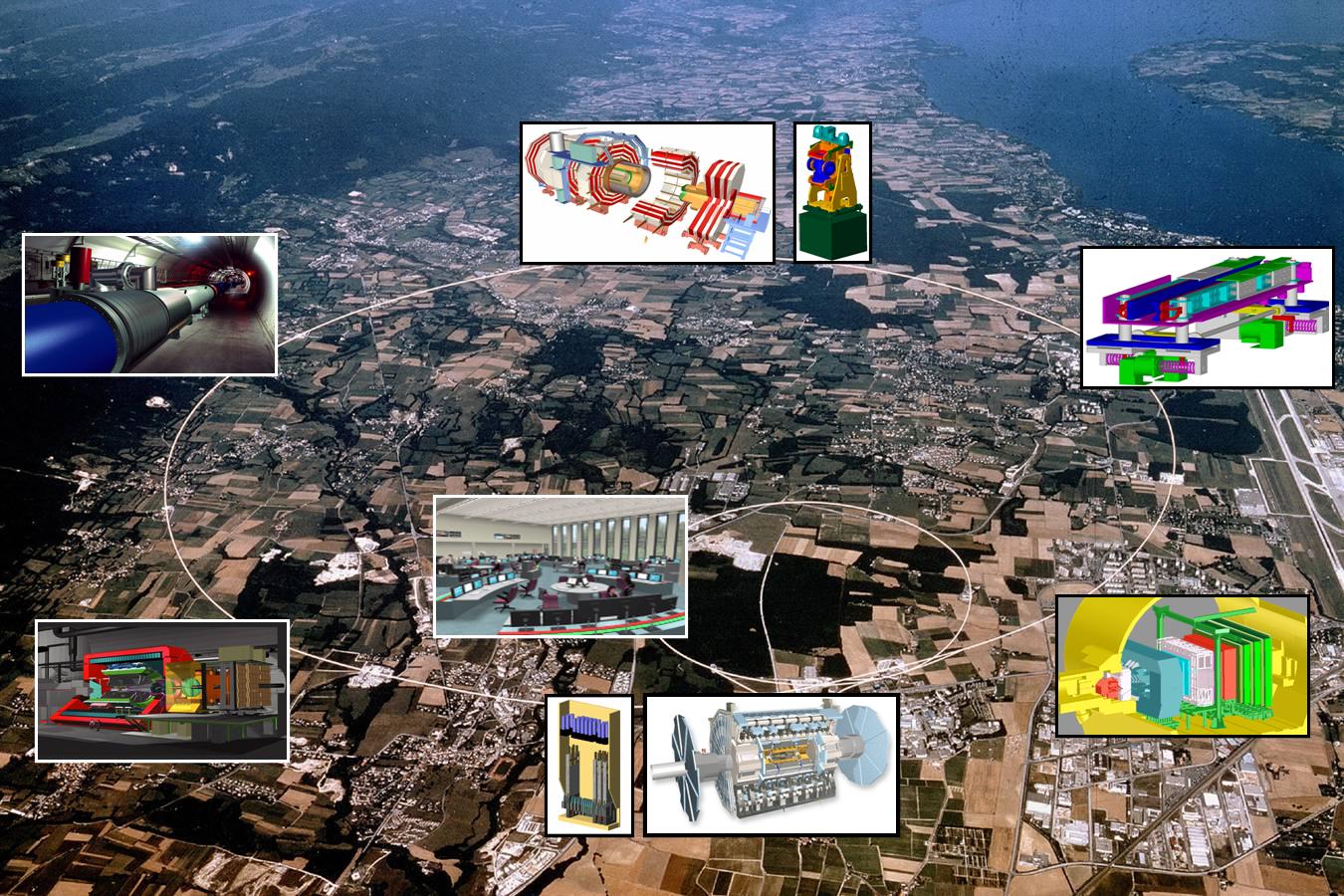
Der Large Hadron Collider (LHC, deutsche Bezeichnung Großer Hadronen-Speicherring)[1] ist ein Teilchenbeschleuniger am Europäischen Kernforschungszentrum CERN bei Genf. In Bezug auf Energie und Häufigkeit der Teilchenkollisionen ist der LHC der leistungsstärkste Teilchenbeschleuniger der Welt. An Planung und Bau waren über 10.000 Wissenschaftler und Techniker aus über 100 Staaten beteiligt, es kooperierten hunderte Universitätslehrstühle und Forschungsinstitute. Die maßgebliche Komponente ist ein Synchrotron in einem 26,7 Kilometer langen unterirdischen Ringtunnel, in dem Protonen oder Blei-Kerne gegenläufig auf nahezu Lichtgeschwindigkeit beschleunigt und zur Kollision gebracht werden. Die Experimente am LHC sind daher Colliding-Beam-Experimente.
Forschungsziele am LHC sind die Erzeugung und genaue Untersuchung bekannter und noch unbekannter Elementarteilchen und Materiezustände. Ausgangspunkt ist die Überprüfung des gegenwärtigen Standardmodells der Teilchenphysik. Besonderes Augenmerk liegt daher auf dem Higgs-Boson, dem letzten bei Betriebsbeginn noch nicht experimentell nachgewiesenen Teilchen des Standardmodells. Darüber hinaus soll der LHC der Suche nach Physik jenseits des Standardmodells dienen, um möglicherweise Antworten auf offene Fragen zu finden. Vier große und zwei kleinere Detektoren registrieren die Spuren der bei den Kollisionen entstandenen Partikel. Durch die große erreichbare Anzahl von Kollisionen pro Sekunde (hohe Luminosität) entstehen enorme Datenmengen. Diese werden mit Hilfe einer ausgeklügelten IT-Infrastruktur vorsortiert. Nur ein kleiner Teil der Daten wird mittels eines eigens aufgebauten, weltumspannenden Computernetzwerks zur Analyse an die beteiligten Institute weitergeleitet.
In den Experimenten wurde ab 2010 ein bisher nicht erreichbarer Energiebereich erschlossen. Ein wesentliches Ergebnis der bisherigen Experimente (Stand: März 2019) ist eine außerordentlich gute Bestätigung des Standardmodells. Mehrere neue Hadronen wurden gefunden, ein Quark-Gluon-Plasma konnte erzeugt werden und erstmals wurde beim Bs0-Meson die CP-Verletzung bei seinem Zerfall in Kaonen und Pionen beobachtet sowie sein extrem seltener Zerfall in zwei Myonen. Auch beim D0-Meson gelang der Nachweis einer CP-Verletzung. Als bislang größter Erfolg gilt der experimentelle Nachweis des Higgs-Bosons. Dies führte zur Verleihung des Nobelpreises für Physik 2013 an François Englert und Peter Higgs.[2]
大型ハドロン衝突型加速器 (おおがたハドロンしょうとつがたかそくき、英: Large Hadron Collider、略称 LHC) は、高エネルギー物理実験を目的としてCERNが建設した世界最大の衝突型円形加速器の名称。スイス・ジュネーブ郊外にフランスとの国境をまたいで設置されている[1]。2008年9月10日[2]に稼動開始した[3]。また、LHC実験はそこで実施されている実験の総称。
LHCは2013年2月から停止していたが、2015年4月5日に改良工事を終え、以前の8兆電子ボルト(8TeV)から13兆電子ボルト(13TeV)の高速エネルギーへ更新して運転を再開した [4]。 13TeVの衝突が2015年5月20日に初めて達成された [5]。
The Large Hadron Collider (LHC) is the world's largest and highest-energy particle collider and the largest machine in the world.[1][2] It was built by the European Organization for Nuclear Research (CERN) between 1998 and 2008 in collaboration with over 10,000 scientists and hundreds of universities and laboratories, as well as more than 100 countries.[3] It lies in a tunnel 27 kilometres (17 mi) in circumference and as deep as 175 metres (574 ft) beneath the France–Switzerland border near Geneva.
First collisions were achieved in 2010 at an energy of 3.5 teraelectronvolts (TeV) per beam, about four times the previous world record.[4][5] After upgrades it reached 6.5 TeV per beam (13 TeV total collision energy, the present world record).[6][7][8][9] At the end of 2018, it entered a two-year shutdown period for further upgrades.
The collider has four crossing points, around which are positioned seven detectors, each designed for certain kinds of research. The LHC primarily collides proton beams, but it can also use beams of heavy ions: lead–lead collisions and proton–lead collisions are typically done for one month per year. The aim of the LHC's detectors is to allow physicists to test the predictions of different theories of particle physics, including measuring the properties of the Higgs boson[10] and searching for the large family of new particles predicted by supersymmetric theories,[11] as well as other unsolved questions of physics.
Le Grand collisionneur de hadrons1, (en anglais : Large Hadron Collider — LHC), est un accélérateur de particules mis en fonction en 2008 et situé dans la région frontalière entre la France et la Suisse entre la périphérie nord-ouest de Genève et le pays de Gex (France). C'est le plus puissant accélérateur de particules construit à ce jour, a fortiori depuis son amélioration achevée en 2015 après deux ans de mise à l'arrêt2. Il est même présenté comme le plus grand dispositif expérimental jamais construit pour valider des théories physiquesNote 1. En 2012, il confirme l'existence du boson de Higgs.
Le LHC a été construit dans le tunnel circulaire (26,659 km de circonférence3) de son prédécesseur, le collisionneur LEP (Large Electron Positron). À la différence de ce dernier, ce sont des protons — de la famille des hadrons — qui sont accélérés pour produire des collisions, en lieu et place des électrons ou des positrons pour le LEP.
Ces protons seront accélérés jusqu'à une énergie de 7 TeV, soit près de 7 500 fois leur énergie de masse. L'énergie totale de deux protons incidents sera ainsi de 14 TeV. Le LHC sera également utilisé pour accélérer des ions lourds comme le plomb avec une énergie totale de collision de 1 150 TeV pour le noyau dans son ensemble soit un peu plus de 2,75 TeV par nucléon qu'il contient.
Huit détecteurs, dont quatre de très grande taille, sont installés sur cet accélérateur, à savoir ATLAS, CMS, TOTEM, LHCb, ALICE, LHCf, MoEDAL et FASER (voir leur description détaillée).
Il Large Hadron Collider (in italiano "Grande Collisore di Adroni"), abbreviato in LHC, è un acceleratore di particelle situato presso il CERN di Ginevra, utilizzato per ricerche sperimentali nel campo della fisica delle particelle.[1]
I componenti più importanti dell'acceleratore sono 1232 magneti superconduttori a bassa temperatura, che hanno un campo intenso circa 8 Tesla[2] . Sono realizzati in lega di niobio e titanio e sono raffreddati alla temperatura di circa 2 K (circa -271 °C), utilizzando elio liquido.
La macchina accelera due fasci che circolano in direzioni opposte, all'interno dei tubi a vuoto. I fasci collidono in quattro punti lungo il percorso, dove il tunnel si allarga per lasciare spazio a grandi vani che ospitano i rivelatori. I quattro principali rivelatori di particelle sono ATLAS, di forma toroidale, il Solenoide compatto per muoni, LHCb, e ALICE, un collisore di ioni. I rivelatori utilizzano tecnologie diverse e operano intorno al punto in cui i fasci collidono. Nelle collisioni vengono prodotte numerose particelle, le cui proprietà vengono misurate dai rivelatori e inviate al centro di calcolo. Tra gli scopi principali degli studi vi è la ricerca di tracce dell'esistenza di nuove particelle.
Dopo un iniziale guasto che ha compromesso l'impianto di raffreddamento e provocato un fermo di circa un anno, LHC ha cominciato la sua campagna sperimentale alla fine del 2009.[3] L'incidente è stato discusso in dettaglio dal fisico Lucio Rossi, all'epoca responsabile dei magneti superconduttori.[4]
Nel 2018 è stata avviata l’attuazione di un progetto di miglioramento delle prestazioni, in particolare di incremento di un fattore 10 della luminosità del fascio (High luminosity LHC project)[5][6].
El Gran Colisionador de Hadrones (LHC; en inglés, Large Hadron Collider) es el acelerador de partículas más grande y de mayor energía que existe y la máquina más grande construida por el ser humano en el mundo.12 Fue construido por la Organización Europea para la Investigación Nuclear (CERN) entre 1998 y 2008 en colaboración con más de 10 000 científicos y cientos de universidades y laboratorios, así como más de 100 países de todo el Mundo.3 Se encuentra en un túnel de 27 kilómetros de circunferencia y a una profundidad de 175 metros bajo tierra debajo de la frontera entre Francia y Suiza, cerca de Ginebra.
Las primeras colisiones se lograron en 2010 a una energía de 3,5 teraelectronvoltios (TeV) por haz, aproximadamente cuatro veces el récord mundial anterior.45 Después de las correspondientes actualizaciones, alcanzó 6,5 TeV por haz (13 TeV de energía de colisión total, el récord mundial actual).6789 A finales de 2018, entró en un período de parada de dos años para nuevas actualizaciones, con el cual se espera posteriormente alcanzar energías de colisión aún mayores.
El colisionador tiene cuatro puntos de cruce, alrededor de los cuales se colocan siete detectores, cada uno diseñado para ciertos tipos de experimentos en investigación. El LHC hace colisionar protones, pero también puede utilizar haces de iones pesados (por ejemplo de plomo) realizándose colisiones de protones de plomo normalmente durante un mes al año. El objetivo de los detectores del LHC es permitir a los físicos probar las predicciones de las diferentes teorías de la física de partículas, incluida la medición de las propiedades del bosón de Higgs10 y la búsqueda de una larga serie de nuevas partículas predicha por las teorías de la supersimetría,11 así como también otros problemas no resueltos en la larga lista de elementos en la física de partículas.
Большо́й адро́нный колла́йдер, сокращённо БАК (англ. Large Hadron Collider, сокращённо LHC) — ускоритель заряженных частиц на встречных пучках, предназначенный для разгона протонов и тяжёлых ионов (ионов свинца) и изучения продуктов их соударений. Коллайдер построен в ЦЕРНе (Европейский совет ядерных исследований), находящемся около Женевы, на границе Швейцарии и Франции. БАК является самой крупной экспериментальной установкой в мире. В строительстве и исследованиях участвовали и участвуют более 10 тысяч учёных и инженеров более чем из 100 стран[1], в том числе из России — 12 институтов и 2 федеральных ядерных центра (ВНИИТФ, ВНИИЯФ).
«Большим» назван из-за своих размеров: длина основного кольца ускорителя составляет 26 659 м[2]; «адронным» — из-за того, что ускоряет адроны: протоны и тяжелые ядра атомов; «коллайдером» (англ. collider — сталкиватель) — из-за того, что два пучка ускоренных частиц сталкиваются во встречных направлениях в специальных местах столкновения — внутри детекторов элементарных частиц[3].
德雷克方程式(英语:Drake equation),又称格林班克方程式(Green Bank equation),是由天文学家法兰克·德雷克于1961年提出的一条用来推测“在银河系内,可以和我们接触的外星智慧文明数量”的方程式[1][2]。
法兰克·德雷克在1961年提出了德雷克方程式,目的不是为了量化外星智慧文明的数量,而是用作在搜寻地外文明计划(SETI)的第一个技术会议时引发科学讨论的方式[3][4]。其公式总结了研究者要考虑以无线电和地球通讯的外星智慧文明数量时,需要考虑的一些概念[3],德雷克公式比较算是估计外星智慧文明,而不是认真的要确定其明确的数量。
有关德雷克公式的批评不是在公式本身,而是估计值中的许多因子都是推测所得的结果,再加上这些因子相乘的效应,其产生的值不确定值太高,因此无法以此公式作出确切的结论。




































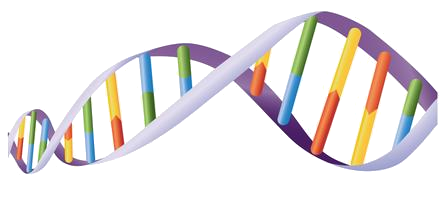

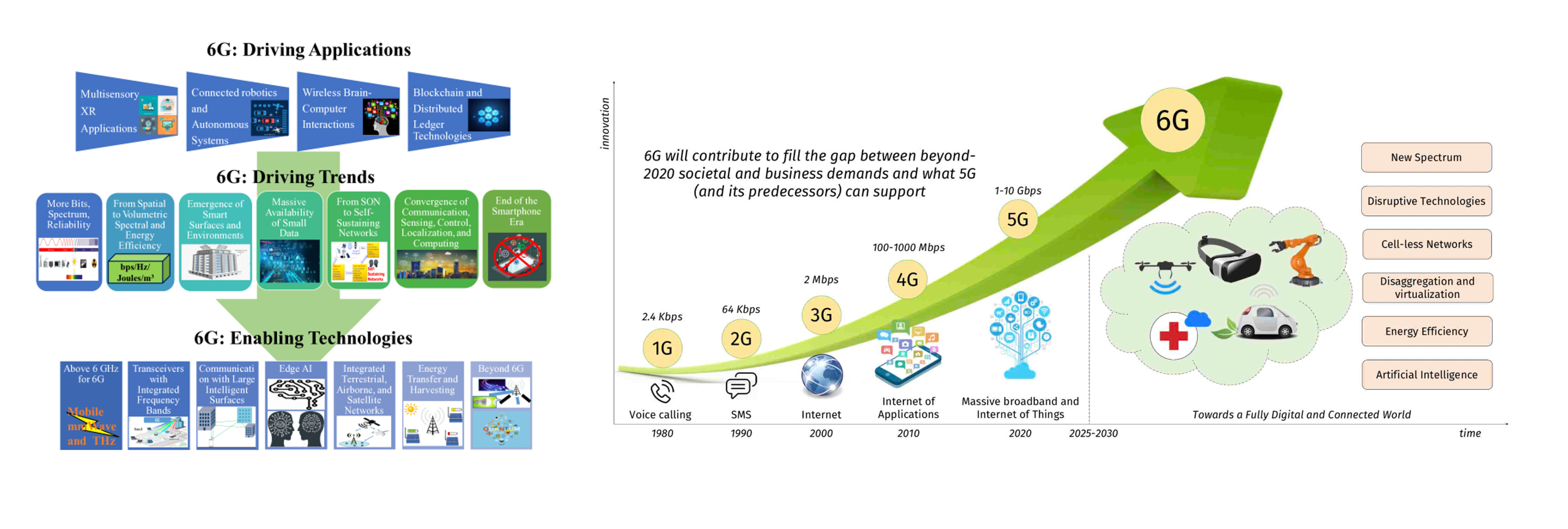












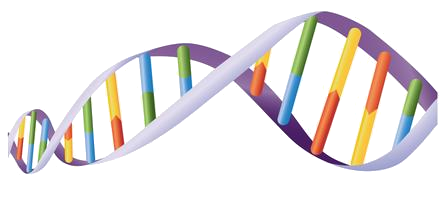

Das Five hundred meter Aperture Spherical Telescope (kurz FAST) ist ein Radioteleskop mit über 500 Meter Durchmesser.
Es befindet sich seit 2011 im Pingtang-Kreis in der Provinz Guizhou im Südwesten von China im Bau. Es soll 2016 fertiggestellt und das größte und genaueste Radioteleskop der Welt werden. Die Kosten sollen 1200 Millionen Yuan betragen (166 Mio. €). Das Gelände wurde unter 30 möglichen Standorten in einer natürlichen Karstdepression gewählt. Die Anlage ist baulich ähnlich dem Arecibo-Observatorium.[1]
Die Anlage ist flexibel aufgebaut und kann die Form der Hauptantenne anpassen, dies ermöglicht Beobachtungen bis zu einer Neigung von bis zu 40° aus dem Zenit. Genutzt wird der Frequenzbereich von 0.3-3.0 GHz, das Teleskop soll eine Genauigkeit von vier Bogensekunden erreichen.[2][3]
500米口径球面射电望远镜(英语:Five-hundred-meter Aperture Spherical radio Telescope,简称FAST)是中国科学院国家天文台的一座射电望远镜,焦比达0.467。FAST位于贵州省平塘县克度镇大窝凼洼地,利用喀斯特洼地的地势而建。其主体工程于2011年开工,2016年落成。FAST是目前世界上最大的单一口径、填充口径(即全口径均有反射面的)射电望远镜[4];并且是世界上第二大的单一口径射电望远镜,仅次于俄罗斯的RATAN-600望远镜(该望远镜为环形)[5]。
500メートル球面電波望遠鏡(中国語: 五百米口径球面射电望远镜、FAST、Five-hundred-meter Aperture Spherical radio Telescope)は、中国南西部の貴州省黔南プイ族ミャオ族自治州平塘県にある、世界最大の電波望遠鏡。中国科学院国家天文台により建設された。中国で「天眼の父」[1]と呼ばれている高名な満州族[2]科学者である同天文台の南仁東(中国語版)が、この計画の責任者を務めた。建設費は約12億元(約185億円)といわれている。
4,450枚(記事によっては4,600枚)の三角形の反射パネルを組み合わせ、固定球面鏡を形成し、望遠鏡直径は本望遠鏡完成まで世界最大であったアレシボ天文台より300メートル大きい500mである。
自然のくぼ地(大窩凼窪地)[3] を利用して作られている[4]。
球面鏡であり、受信機は500mの鏡面全体をカバーすることはできず、有効直径として機能するのは300m分である。地面に固定されているため、観測可能範囲は天頂から40度の範囲までである。観測周波数は0.3-5.1GHzを、指向精度は4秒角を見込んでいる[5]。
当施設建設に際し、地上からの電波や光の干渉を取り除く為、周囲5kmに渡る「緩衝圏」と称すエリアと、その周囲に観光客の為の公園(博物館や宇宙をテーマにしたホテル及びレセプション施設が入る。総工費15億元(約248億円)と、望遠鏡施設より高い)が建設予定となっており、その実行の為、約1万人(報道によっては約9,000人)とも言われる住民を移住させる方針が発表され、国営メディアの新華社は「近隣の人々はその幸運をうらやましがっている。彼らは宇宙人に感謝すべきだ」と報じるも当局の強引なやり方に対する地元住民による訴訟も起きている[6]。
The Five-hundred-meter Aperture Spherical radio Telescope (FAST; Chinese: 五百米口径球面射电望远镜), nicknamed Tianyan (天眼, lit. "Sky Eye" or "The Eye of Heaven") is a radio telescope located in the Dawodang depression (大窝凼洼地), a natural basin in Pingtang County, Guizhou Province, southwest China.[1] It consists of a fixed 500 m (1,600 ft) diameter dish constructed in a natural depression in the landscape. It is the world's largest filled-aperture radio telescope,[2] and the second-largest single-dish aperture after the sparsely-filled RATAN-600 in Russia.[3][4]
It has a novel design, using an active surface made of metal panels that can be tilted by a computer to help change the focus to different areas of the sky.[5] The cabin containing the feed antenna suspended on cables above the dish is also moved using a digitally-controlled winch by the computer control system to steer the instrument to receive from different directions.
Construction on the FAST project began in 2011 and it achieved first light in September 2016.[6] It is currently undergoing testing and commissioning.[7] It observes at wavelengths of 10 cm to 4.3 m.[3]:11[8]
The telescope made its first discovery of two new pulsars in August 2017, barely one year after its first light.[9] The new pulsars PSR J1859-01 and PSR J1931-02, which are also referred to as FAST pulsar #1 and #2 (FP1 and FP2), were detected on 22 and 25 August and are 16,000 and 4,100 light years away, respectively. They were independently confirmed by the Parkes Observatory in Australia on 10 September. The telescope had discovered 44 new pulsars by September, 2018.[10][11][12]
Le radiotélescope sphérique de cinq cents mètres d'ouverture (chinois traditionnel : 五百米口徑球面射電望遠鏡 ; chinois simplifié : 五百米口径球面射电望远镜 ; pinyin : Wǔbǎi mǐ kǒujìng qiúmiàn shèdiàn wàngyuǎnjìng), abrégé en anglais FAST pour Five-hundred-meter Aperture Spherical Radio Telescope1, est un radiotélescope situé dans un bassin naturel du comté de Pingtang, dans le Guizhou, dans le sud-ouest de la Chine. Le radiotélescope est mis en service le 25 septembre 2016. Il est le deuxième plus grand radiotélescope au monde après le RATAN-600 en Russie et le plus grand radiotélescope à un seul appareil, avec une sensibilité égale à environ trois fois celle du radiotélescope d'Arecibo.
Il FAST, acronimo di Five hundred meter Aperture Spherical Telescope ("Telescopio sferico con apertura di cinquecento metri"; in cinese: 五百米口径球面射电望远镜), è un radiotelescopio collocato nel sudovest della Cina, posizionato nella concavità di un bacino naturale (cinese: 大窝凼洼地; letteralmente: "depressione Da Wo Dang") nella contea di Pingtang, nella provincia del Guizhou.
Il progetto per la costruzione del FAST ha avuto inizio nel 2011 ed è stato completato a settembre 2016. Attualmente è il radiotelescopio più grande e più sensibile al mondo, tre volte più sensibile del radiotelescopio dell'Osservatorio di Arecibo.[1][2] Il suo costo previsto di 700 milioni di yuan[3] (circa 110 milioni di dollari statunitensi o 100 milioni di euro) è lievitato fino a raggiungere il valore finale di 1,2 miliardi di yuan (circa 160 milioni di euro)[4].
El Telescopio esférico de quinientos metros de apertura (FAST) (chino: 五百米口径球面射电望远镜) o Tianyan (en chino: 天眼: 天眼) es un radiotelescopio localizado en una cuenca natural de Da Wo Dang (en chino: 五百米口径球面射电望远镜: 大窝凼洼地), en el Condado de Pingtang, provincia Guizhou, al suroeste de China.2
La construcción del proyecto FAST empezó en 2011, y logró su primera luz el 25 de septiembre de 2016. Está actualmente bajo pruebas y puesta en servicio. Es el segundo radiotelescopio más grande del mundo después del ruso RATAN-600, el cual tiene una apertura de baja densidad de llenado.34 Su presupuesto fue de 700 millones de yuan5 (alrededor 110 millones dólares) y su costo final fue de 1 200 millones de yuan6 (unos 180 millones de dólares).
FAST (кит. 五百米口径球面射电望远镜, англ. Five hundred meter Aperture Spherical Telescope — «Сферический радиотелескоп с пятисотметровой апертурой») — радиотелескоп на юге Китая в провинции Гуйчжоу[2][3]. На строительство радиотелескопа было затрачено более 185 миллионов долларов[4].
После окончания строительства в 2016 году и сдачи в эксплуатацию FAST стал самым большим в мире радиотелескопом с заполненной апертурой, его диаметр — 500 метров. Существует радиотелескоп с незаполненной апертурой большего диаметра — российский 576-метровый радиотелескоп РАТАН-600[5].
Радиотелескоп позволит учёным изучать формирование и эволюцию галактик, тёмную материю, исследовать объекты эпохи реионизации и решать другие научные задачи[6].
